About
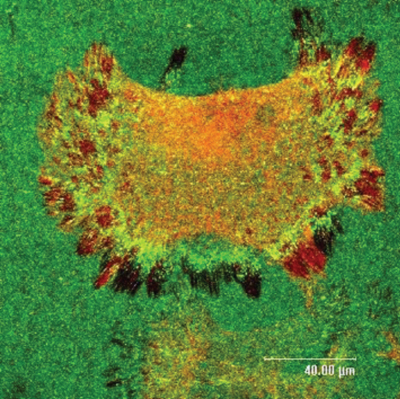
We are interested in cell–biomaterials interaction, and more specifically, on the dynamic formation of the provisional extracellular matrix (ECM) – the thin protein layer that cells recognize, produce, and remodel at the materials interface.
We aim to learn how this process affects the biocompatibility of materials, and if it can be controlled by engineering the surface properties of materials. For this purpose, we perform systematic studies in the following directions:
Remodelling of ECM proteins at cell-biomaterials interface
Upon adsorption at material interfaces, proteins may assemble spontaneously and this interaction has significant consequences for their biological activity. The cells can also actively rearrange these proteins presumably as an attempt to organize a provisional ECM. We anticipate that materials that bind proteins loosely will support the arrangement of a provisional ECM, while stronger binding provokes its degradation, i.e. their proteolytic remodeling. ECM remodelling is a fundamental proces that occurs in various physiological and pathological conditions, such as normal development, wound healing and angiogenesis, but also in atherosclerosis, fibrosis, ischemic injury and cancer. It is dynamic and consists of two fundamental processes: assembly and degradation. Although matrix remodelling is a subject of extensive biomedical research, the way it is related to the biocompatibility of materials is poorly understood and is therefore a hot topic of our research.
ECM organization at the biomaterial interface depends on the allowance of cells to rearrange adsorbed matrix proteins – a process strongly dependent on proper functioning of integrin receptors. We anticipate that materials that bind proteins loosely will support the arrangement of a provisional ECM, while stronger binding provokes its degradation.
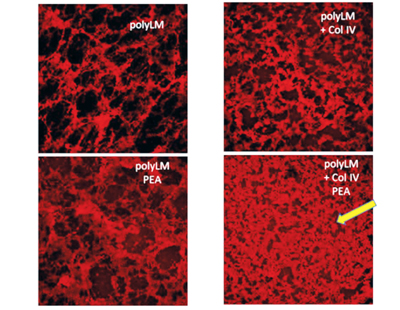
Biomaterials surface-driven assembly of ECM proteins at the nanoscale
Upon adsorption at material interfaces, proteins may assemble spontaneously and this interaction has significant consequences for their biological response. Recently we have employed distinct silane-inspired chemistries and polymer compositions to create model substrates with tailored densities of -OH, -COOH, -NH2 and -CH3 groups, thus varying the chemistry, charge and hydrophilic/ hydrophobic balance. In a series of communications combining AFM and other nanoindentation techniques, we have described a novel phenomenon of substratum-driven protein assembly depicting the fate of various matrix proteins such as fibronectin, collagen IV, vitronectin and fibrinogen at the above model biomaterials interfaces.
Specifically, we show that by varying the density of chemical functions one can tailor both the assembly and degradation of proteins. Following those findings we aim to control ECM remodelling by engineering specific material properties. Understanding the behavior of ECM proteins on flat biomaterials interface further boosts an important bioengineering target – the biohybrid organ technologies based on two-dimensional protein layers that mimic the arrangement of the natural basement membrane.
Development of artificial basement membrane
Understanding the behavior of ECM proteins on flat biomaterials interface further boosts an important bioengineering target – the biohybrid organ technologies based on two-dimensional protein layers that mimic the arrangement of the natural basement membrane. With this project we aim to develop a synthetic basement membrane (BM) to be used as a supportive lining for cellularized implants, with specific focus on the design of a bioengineered blood vessel. Taking advantage of the self-assembly properties of the two principal components of the BM, laminin and collagen IV, composite matrices of these molecules are produced by mixing them before or during the polymerization of laminin under acidic conditions.
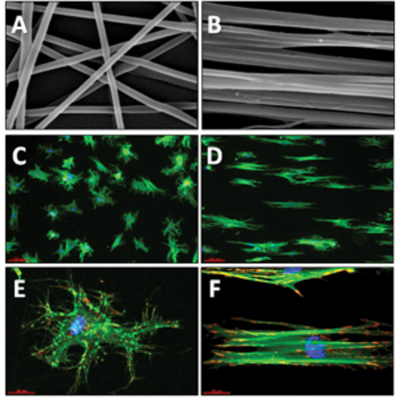
Selected composites will be deposited on scaffolds produced using electronspun nanofibers preferentially made of polyethyl acrilate (PEA), which additionally favour networking of laminin and collagen IV. The resemblance to natural BM will be evaluated in terms of their morphological features and ability to properly induce the formation of biomimetic monolayers of endothelial cells. This project is driven involving joint efforts of our Lab and this of Prof Tatiana Coelho-Sampaio’s from the Federal University of Rio de Janeiro, Brazil.
Electrospinning of nanofibers from natural and synthetic polymers for guiding cellular behaviour
In solution, proteins can form structures of various shapes, including fibers with a diameter of only a few nanometers and with lengths up to centimeters. A fascinating possibility to mimic similar ECM structures is to engineer protein-like or matrix protein-containing nanofibers via electrospinning technology. For this purpose we are developing electrospun nanofibers from natural (e.g., fibrinogen) and synthetic polymers (e.g. PLA, PEA) in order to direct the desired cellular response via spatially organized cues (e.g. fiber size and geometrical organization) as well as by tailoring their chemical and mechanical properties.
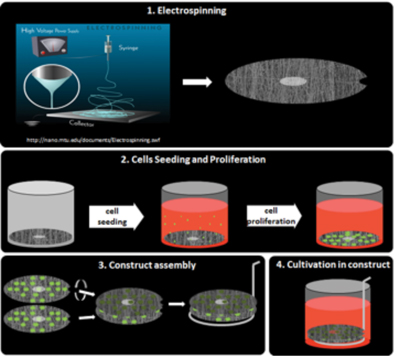
Nanofibers-based 3D constructs for culturing of stem cells with spatially organized stimuli
Examining hierarchical biology in only two dimensions (i.e., cells confined to a monolayer) is in most cases insufficient as cells typically exhibit unnatural behavior if excised from native three-dimensional (3D) tissues. Therefore, within the European FIBROGELNET project (under our coordination) we are developing 3D biohybrid constructs that combine the structural and biological properties of electrospun nanofibers with the optimized mechanical properties of specific hydrogels in order to provide stem cells with relevant spatial orientation in three dimensions.
Creating dynamic stem cell niches using stimuli-responsive biomaterials
In addition to engineering the spatial configuration of cellular microenvironments, we are also interested in addressing the dynamic (i.e., temporal) aspects of the stem cell niche. To do that we take advantage of stimuli-responsive polymers to obtain control over an artificial cell-adhesive environment via dynamically altering either cell-cell (using cadherin-like ligands) or cell-matrix (using ECM proteins) interactions. By modulating the strength of adhesive protein-to-substratum interactions we aim to control the stem cell adhesive machinery, and which allows us to mimic the dynamic conditions of the stem cell niche.
Reversible attachment/detachment of human mesenchymal stem cells from thermo-responsible PNIPAM substrata: Cells were cultured at 37ºC for 5 h on PNIPAM (A) and left to detach at room temperature for 2 hours (B), then switched again to 37ºC overnight (C)
Projects
EU-funded projects
STRUCTGEL Nanostructured Gel for Cellular Therapy of Degenerative Skeletal Disorders | EURONANOMED | George Altankov |
FIBROGELNET Network for Development of Soft Nanofibrous Construct for Cellular Therapy of Degenerative Skeletal Disorders | FP7-PEOPLE-2012-IAPP | George Altankov |
National projects
Materiales que inducen la fibrilogénesis de la fibronectina para producir microambientes sinérgicos en los factores de crecimiento | I+D-Investigación fundamental no orientada | George Altankov |
MYOREM Remodelación por mioblastos de la matriz extracelular en la interfaz celula-biomaterial (2016-2018) | MINECO, Retos investigación: Proyectos I+D | George Altankov |
MYOHEAL Muscle regeneration after injury. Engineered biodegradable ion–loaded scaffolds to promote muscle regeneration (2015-2017) | MINECO, MAT 2015 – 69315 –C3 | George Altankov |