ABOUT
Tissues in our body can be extremely soft such as breast or brain, or very stiff such as bone. Cells in our body constantly interact mechanically with such tissues, exerting, transmitting, withstanding, and detecting forces. This mechanical interaction with the environment regulates how cells proliferate, differentiate, and move, and regulates development, tumorigenesis or wound healing.
Our research aims at unraveling – and re-engineering – the molecular mechanisms by which cells detect and respond to mechanical stimuli like forces or tissue rigidity, triggering downstream cell responses.
Just like biochemical stimuli initiate signaling cascades, mechanical forces affect the links and conformation of a network of molecules connecting cells to the extracellular matrix. This molecular and cellular response to force constitutes the phenomenon of mechanotransduction.
To study mechanotransduction, we combine biophysical techniques like magnetic and optical tweezers, Atomic Force Microscopy, traction microscopy, and microfabricated force sensors with molecular biology, advanced optical microscopy, and theoretical modelling.
Sensing the environment: Using this multi-disciplinary approach, we have unveiled a molecular mechanism that cells employ to detect and respond to the rigidity of their environment, which could be crucial in breast tissue and breast cancer (Elosegui-Artola et al., 2016 Nat. Cell Biol., and Elosegui-Artola et al. 2014, Nature Mater.). This mechanism is mediated by what is known as a “molecular clutch”: in a surprising analogy with a car engine, cells can be understood as a molecular network that can engage and disengage from its environment, just as the clutch of a car. This affects force transmission from the environment to cells, and also within different cell components. We are also expanding on the idea of the molecular clutch, to explore how cell molecular engines sense not only mechanical rigidity, but other important parameters from their environment: for instance, the composition and distribution of ligands in the extracellular matrix, or other cells. In this regard, we uncovered that this concept can explain how cells sense the spatial distribution of ligands in the extracellular matrix (Oria et al., Nature 2017). We have also demonstrated that cell-cell force transmission, mediated by a molecular clutch, is essential for cells to sense gradients in stiffness (Sunyer et al., Science 2016, in collaboration with the group of Xavier Trepat).
Nuclear mechanotransduction: Forces applied to cells are transmitted all the way to the cell nucleus, where they affect its function. We are studying how this force transmission affects the dynamics of transcriptional regulators, such as YAP (Elosegui-Artola et al., 2017, Cell), and how this affects cell function.
The membrane as a mechanosensor: Due to its mechanical properties, the plasma membrane itself can respond to forces and act as a mechanosensor. Recently, we have shown that cell membranes can use purely physical principles to adapt their shape in response to mechanical forces (Kosmalska et al., 2015, Nat. Commun.). We are currently studying how cells harness this physical membrane behavior to respond to signals from their environment.
Ultimately, when we determine the molecular mechanisms that communicate cells with their environment, we will understand how forces determine development when things go right, and tumor formation when they go wrong.
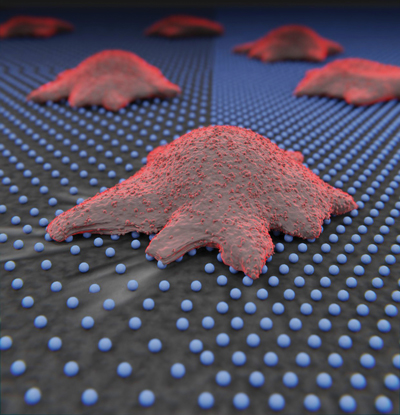
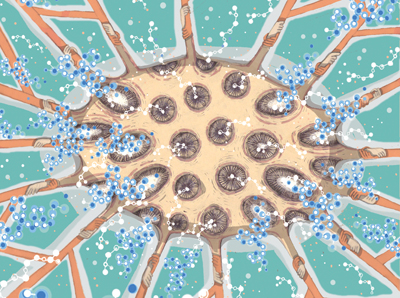
Video: How tissue stiffness activates cancer
STAFF
The following is a list of the current staff members of the research group:
PROJECTS
NATIONAL PROJECTS | FINANCER | PI |
---|---|---|
MECNUC · Estudio del control mecánico de la localización nuclear de proteínas (2020-2023) | MINECO Retos investigación: Proyectos I+D | Pere Roca-Cusachs |
BLOCMEC Development of small molecules to block mechanotransduction for pancreatic cancer therapy (2021-2023) | MICIU, Proyectos Pruebas de Concepto | Pere Roca-Cusachs |
INTROPY INhibiting mechanoTRansduction for Oncology theraPY (2021-2023) | ACCIO, Tecniospring Industry | Mamatha Nijaguna |
INTERNATIONAL PROJECTS | FINANCER | PI |
---|---|---|
MECHANOCONTROL · Mechanical control of biological function (2017-2021) | European Commission, FET Proactive | Pere Roca-Cusachs |
TALVIN · Inhibiting mechanotransduction for the treatment of pancreatic cancer (2018-2021) | European Commission, FET Innovation Launchpad | Pere Roca-Cusachs |
MECHANOSITY Mechanical regulation of cellular behaviour in 3D viscoelastic materials (2019-2022) | European Commission, MARIE CURIE | Alberto Elosegui |
PRIVATELY-FUNDED PROJECTS | FINANCER | PI |
---|---|---|
Mech4Cancer · Enabling technologies to map nuclear mechanosensing: from organoids to tumors (2020-2023) | Obra Social La Caixa Health Research Call | Pere Roca-Cusachs |
Understanding YAP-mediated mechanotransduction in pancreatic cancer (2020-2023) | Fundació La Marató de TV3 | Pere Roca-Cusachs |
Understanding and measuring mechanical tumor properties to improve cancer diagnosis, treatment, and survival: Application to liquid biòpsies (2017-2022) | Obra Social La Caixa | Pere Roca-Cusachs |
FINISHED PROJECTS | FINANCER | PI |
---|---|---|
Desarrollo de una terapia innovadora para el tratamiento de los tumores sólidos mediante la inhibición de la mecanotransducción (2018-2020) | MINECO, Subprograma Retos-Colaboración | Pere Roca-Cusachs |
Understanding and measuring mechanical tumor properties to improve cancer diagnosis, treatment, and survival: Application to liquid biopsies (2017-2020) | Obra Social La Caixa | Pere Roca-Cusachs |
IMREG El sistema acoplado entre integrinas y proteínas adaptadoras como regulador mecánico del comportamiento celular (2016-2020) | MINECO, Proyectos I+D Excelencia![]() | Pere Roca-Cusachs |
MECHANOMEMBRANE Redes mecanoquímicas en la membrana plasmática (2017-2018) | MINECO, Subprograma Estatal de Generación de Conocimiento “EUROPA EXCELENCIA” | Pere Roca-Cusachs |
Stromal stiffness in tumor progression (2014-2017) | Fundació La Marató de TV3 | Pere Roca-Cusachs |
MECBIO Red de Excelencia en Mecanobiología (2014-2016) | MINECO, Subprograma Estatal de Generación de Conocimiento “REDES DE EXCELENCIA” | Pere Roca-Cusachs |
Inhibiting mechanostransduction as a novel therapy in the treatment of solid tumors (2017-2018) | Obra Social La Caixa | Pere Roca-Cusachs |
PUBLICATIONS
Click here for a list of publications by Pere Roca-Cusachs with IBEC affiliation.
Click here for a full list of publications including those affiliated to other organisations.
EQUIPMENT
- Confocal Microcopy
- Traction Microscopy
- Live cell fluorescence microscopy
- Cell stretching
- Cell culture
- Magnetic Tweezers
- Atomic Force Microscopy
- Surface Micro/Nano-patterning
- Optical tweezers
COLLABORATIONS
- Dr. Nils Gauthier
Mechanobiology Institute, Singapore - Prof. Miguel Ángel del Pozo
Centro Nacional de Investigaciones Cardiovasculares (CNIC), Madrid - Prof. Marino Arroyo
UPC, Barcelona - Prof. Ada Cavalcanti
University of Heidelberg, Germany - Satyajit Mayor
National Centre for Biological Sciences, Bangalore, India - Sergi Garcia-Manyes
King’s College, London, UK - Louise Jones
Barts Cancer Institute, London, UK - Aránzazu del Campo
INM Saarbrücken, Germany - Patrick Derksen
UMC Utrecht, the Netherlands - Johanna Ivaska
University of Turku, Finland - Jacco van Rheenen
Netherlands Cancer Institute, Netherlands - Isaac Almendros and Ramon Farré
UB, Barcelona - Marc Martí-Renom
CNAG, Barcelona - Marc Güell
UPF, Barcelona - Francisco Real
CNIO, Madrid - Jonas Ries
Max Perutz labs, Vienna
Clinical collaborations
- University Medical Centre Utrecht
- Vall d’hebron Institute of Oncology
NEWS
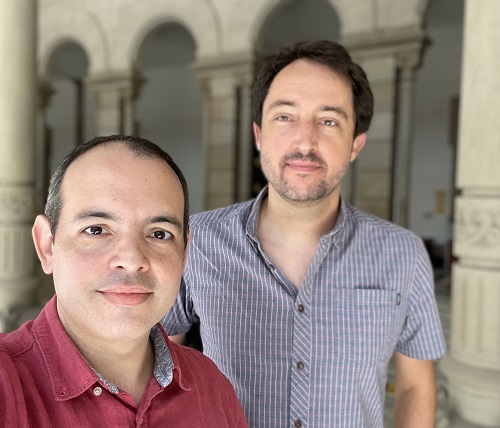
Cellular push and pull, a key to the body’s response to processes such as cancer
Researchers, led by Pere Roca-Cusachs at the Institute for Bioengineering of Catalonia (IBEC) discover how force dynamics affect cells, and living tissues. The results give an insight into the critical mechanical processes that occur in different diseases such as cancer.
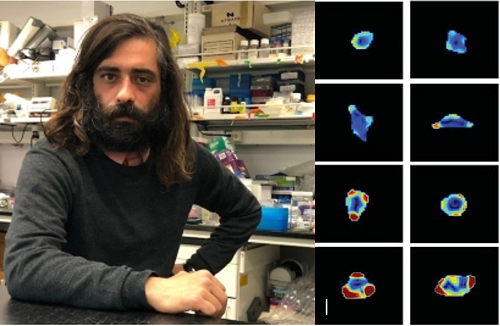
Awarded a thesis on mechanobiology at IBEC
Roger Oria wins the XXIV Doctors’ Senate Award of the University of Barcelona (UB) for his thesis on mechanobiology, an emerging discipline that can help identify new tools to stop pathologies associated with tissue stiffness, such as cancer.
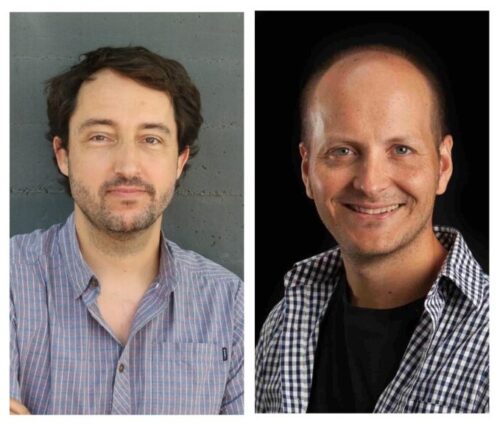
Bioengineering against cancer
Xavier Trepat and Pere Roca-Cusachs, group leaders at IBEC, appear on the journal “ABC” explaining their research project that they will carry out thanks to funding from “la Caixa” as part of the «Health Research Call ».
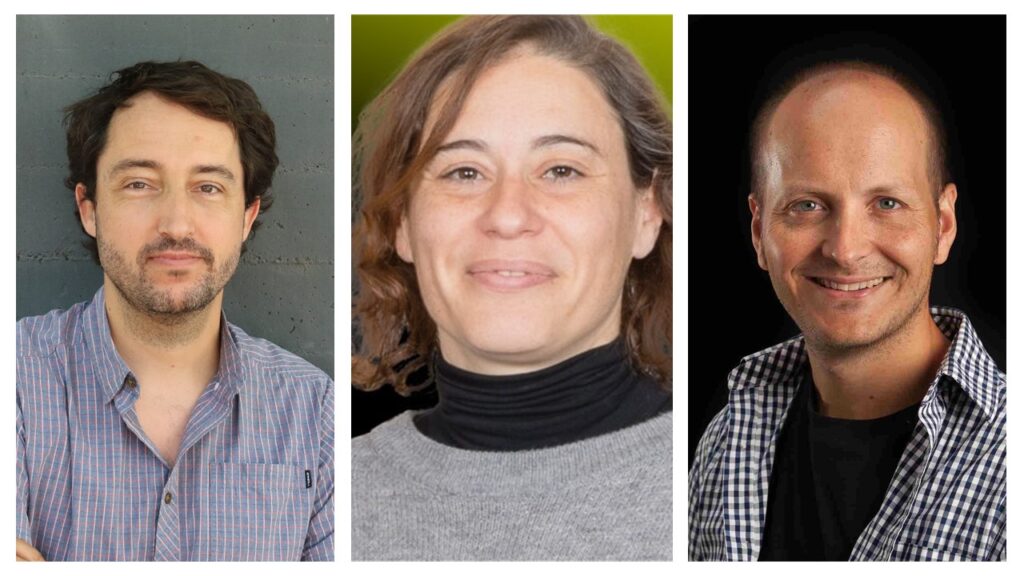
Bioengineering against cancer: IBEC researchers receive funding from La Caixa
IBEC researchers Elena Martínez, Xavier Trepat and Pere Roca-Cusachs aim to understand the processes that promote metastasis in colorectal cancer using innovative bioengineering tools, such as bioprinting and microscopy capable of revealing forces at the cellular level. The results will be translated into a device that will recreate the tumor environment from cancer cells derived from patients, as well as a new technology that will allow to visualize how physical forces affect the nuclei of metastatic cells.
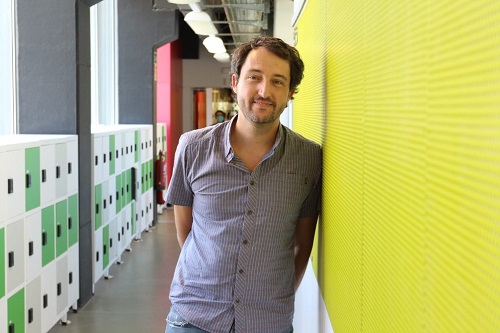
Pere Roca-Cusachs joins the European elite club in biology
Pere Roca-Cusachs, group leader at the Institute for Bioengineering of Catalonia (IBEC) and associate professor at the Faculty of Medicine of the University of Barcelona (UB), has been chosen to join the European Molecular Biology Organization (EMBO) , a prestigious network that brings together some of the most brilliant researchers in the world. Roca-Cusachs is a pioneer in Europe in the mechanobiology field and in the study of how physical forces affect diseases such as cancer.
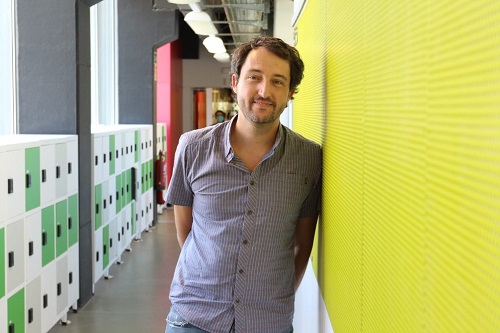
Pere Roca-Cusachs elected new EMBO member
Pere Roca-Cusachs, principal investigator at IBEC, is the only Catalan chosen this year to join the prestigious European Molecular Biology Organization (EMBO).
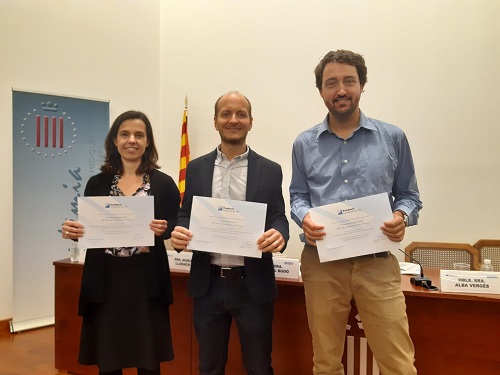
IBEC recieves funding for three projects thanks to la Marató 2018
Three IBEC projects have been selected to receive funding from “La Marató 2018: Against Cancer.” One of the projects is led by the researcher Pere Roca-Cusachs and the other two are co-led by the researchers Xavier Trepat and Núria Montserrat. The awarding ceremony took place on October 30 in the Auditorium of the Academy of Medical and Health Sciences of Catalonia and the Balearic Islands. In this edition, over the 188 evaluated projects, 43 have been selected by an international committee of experts in cancer based on their excellence, methodology and relevance. La Marató de TV3, together with Catalunya Ràdio, broadcasts its annual telethon to raise funds for scientific research into various diseases with a different theme each year.
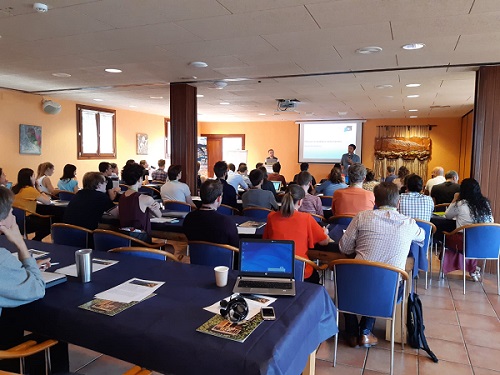
Great success of the Mechanobiology of Cancer Summer School 2019 organised by the Mechano·Control project
More than 60 people attended the “Mechanobiology of Cancer Summer School 2019” organised by IBEC as the center is in charge of coordinating the Mechano·contorl project. The summer school was held in Prullans, a tiny village located at the Catalan Pyrinees between 17 and 21 of September. The event was a great success both in participation and scientific level. The aim of the summer school was to provide training on mechanobiology, and specifically its application to breast cancer, and promote interactions between professionals of the field. The school included lectures as well as practical workshops in different techniques and disciplines, ranging from modelling to biomechanics to cancer biology. The Mechano·Control project, coordinated by Pere Roca-Cusachs, principal investigator of the IBEC is the largest European project coordinated by the IBEC to date.
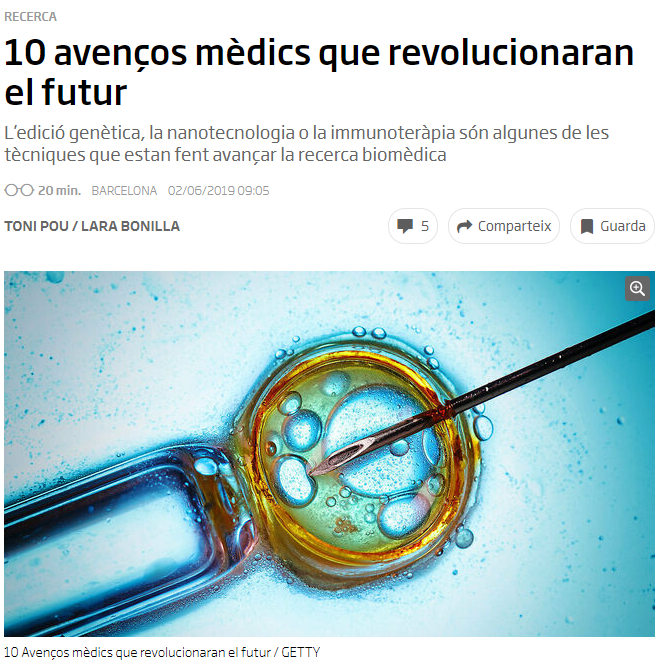
10 medical advances that will revolutionize the future
Nuria Montserrat and Pere Roca-Cusachs interviewed in the article “10 scientific advances that will revolutionize the future” of the newspaper Ara, talking about 3D tissue printing and mechanobiology.
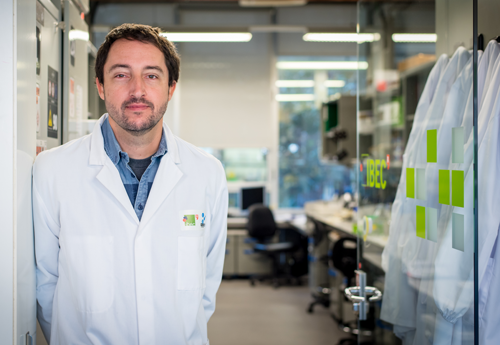
Pere Roca winner of EBSA Young Investigator’s Prize
Pere Roca-Cusachs, group leader at IBEC and assistant professor at the University of Barcelona, has won the 2019 Young Investigator Prize for his contributions to the field of mechanobiology. The award is given by the European Biophysical Societies Association (EBSA). EBSA association grants this prize every two years. The last winner of the prize was Philipp Kukura from the University of Oxford in the UK in 2017. The prize recognises an investigator who has defended his thesis 12 years ago or less across Europe and awards him with 2000€ and a medal as well as be expected to contribute an article to the European Biophysics Journal.